Tracheophytes are distinguished from bryophytes by their highly developed vascular systems, which facilitate the transport of water and nutrients to all parts of the plant. This vascularization adaptation has allowed tracheophytes to become more fully terrestrial than bryophytes, which are still dependent upon moist environments for many reproductive and nutritive functions, as discussed in Bryophytes. Tracheophytes can be broken down into three classes: ferns, gymnosperms, and angiosperms. Ferns are the least evolved of the tracheophytes; they have vascular systems, and specialized leaf and root structures, but are still dependent on moist environments for reproduction. Gymnosperms (coniferous plants) and angiosperms (flowering plants), known together as the seed plants, have evolved reproductive processes that are independent of water. In addition, tracheophyte seed plants all produce embryos that are encased in tough coats. These seed coats prevent desiccation in a terrestrial environment and protect the seed until conditions are favorable for growth. Angiosperms can be further classified as monocots and dicots, depending on their embryonic development and other factors.
Vascular Tissues
Tracheophytes are characterized by the presence of vascular tissue, composed of specialized conductive cells that create "tubes" through which materials can flow throughout the plant body. These vessels are continuous throughout the parts of the plant, allowing for the efficient and controlled distribution of water and nutrients. In addition to this transport function, vascular tissues also provide a measure of support to the plant, contributing to tracheophytes' ability to grow much larger and higher than nonvascularized plants. The two types of vascular tissue are xylem (dead cells) and phloem (living cells). Roots and root hairs, through which the bulk of water and minerals enter the plant body, are also integral to the vascular system of tracheophytes.
Xylem and Phloem
Xylem consists of a "pipeline" of dead cells arranged end to end for water and mineral transport. When the cells that form xylem die at maturity, the nucleus and cytoplasm disintegrate, leaving a hollow tunnel. The leftover cell walls are very thick and provide support for the plant; the cavities inside provide a passage through which fluids can move. The xylem carries water and dissolved minerals upward from the roots through the stem and leaves of the plant. In larger seed plants, xylem cells are specialized into vessel elements and tracheids. Vessel elements are found in flowering plants (angiosperms), and are wider and better at conducting water than the tracheids of conifers (gymnosperms).
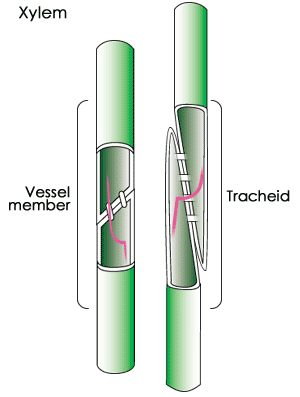
Unlike xylem, the cells that make up phloem are living at maturity and can carry materials both up and down the plant body. Phloem is comprised of sieve elements, which are arranged end to end to form passageways, and companion cells, which are closely associated with the sieve elements even though their exact function is unknown. Mature companion cells have both a nucleus and cytoplasm, while the mature sieve elements contain only cytoplasm; for this reason it is thought that the nuclei of companion cells may control the activities of neighboring sieve elements. Phloem is responsible for distributing the products of photosynthesis, such as amino acids and carbohydrates, from the leaves to the rest of the plant.

Roots
Like the stem, the roots of a tracheophyte contain vascular bundles composed of xylem and phloem. Although the relative position of the two tissues is different, the transport system within the roots is continuous with that in the shoot, allowing for the efficient movement of water and nutrients up and down the plant body. The roots draw water and minerals from the soil and pass them upward to the stem and leaves. They are also responsible for storing the plant's organic nutrients, which are passed downward from the leaves through the phloem. Radiating from the roots are a system of root hairs, extend from the surface of the root and vastly increase the absorptive surface are of the roots. For more information on roots and root hairs, see Roots, Plant Strucutres
Growth in Vascular Plants
Vascular plants undergo two kinds of growth, called primary and secondary growth. Primary growth occurs in the apical meristems, located at the tip of both root and shoot, and is mainly a growth of vertical length. The meristems are regions of rapid mitotic division, almost a cell-making factory. When a cell divides, one of its offspring moves down into the plant body where it elongates, and the other remains in the meristem to divide again.