Statement of Kepler's Second Law
Kepler's Second Law can be stated in several equivalent ways:
- If we draw a line from the sun to the planet in question (a radius), then as the planet moves around in its orbit it will sweep out some area $A_1$ in time $t$. If we consider the planet elsewhere on its orbit, then over the same time interval $t$ its radius will sweep out another area, $A_2$. Kepler's Second Law states that $A_1 = A_2$. This law is often referred to as the "law of equal areas."
- Alternatively, any two radial lines between the sun and the elliptical orbit of a planet form some area (for convenience let us again call this $A_1$). The points where these radii intersect the orbit are labeled $p_1$ and $q_1$. We then choose two more radial lines that form another area $A_2$ that is equal in size to $A_1$ and label the points where these radii intersect $p_2$ and $q_2$. Then Kepler's Second Law tells us that the time taken for the planet to pass between points $p_1$ and $q_1$ is equal to the time taken to pass between points $p_2$ and $q_2$.
Keplers Second Law means that the closer a planet is to the sun, the faster it must be moving on its orbit. When the planet is far away from the sun, it only has to move a relatively small distance in order to sweep out a large area. However, when the planet is close to the sun it must move a lot further in order to sweep out an equal area. This can be seen most clearly in .
Kepler's Second Law and Conservation of Angular Momentum
Kepler's Second Law is an example of the principle of conservation of angular momentum for planetary systems. We can make a geometrical argument to show how this works.
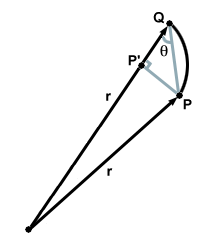
Consider two points $P$ and $Q$ on the orbit of a planet, separated by avery small distance. Suppose that it takes a small time $dt$ for the planet to move from $P$ to $Q$. Because the line segment $\vec{PQ}$ is small, we can make the approximation that it is a straight line. Then $\vec{PQ}$, being the infinitesimal distance $dx$ over which the planet moved in time $dt$, represents the average velocity of the planet over that small range. That is $\vec{PQ} = \vec{v}$. Now consider the area swept out in this time $dt$. It is given by the area of the triangle $SPQ$, which has height $PP'$ and base $r$. But it is also clear from that $PP' = |PQ|\sin\theta$. Thus the area swept out per time $dt$ is given by: \begin{equation} \frac{dA}{dt} = \frac{1}{2}\times r \times |PQ| \times \sin\theta = \frac{rv\sin\theta}{2} \end{equation} But Kepler's Second Law asserts that equal areas must be swept out in equal time intervals or, expressed differently, area is swept out at a constant rate ($k$). Mathematically: \begin{equation} \frac{dA}{dt} = k \end{equation} But we just this value: \begin{equation} \frac{dA}{dt} = k = \frac{rv\sin\theta}{2} \end{equation} Angular momentum is given by the expression: \begin{equation} \vec{L} = m(\vec{v} \times \vec{r}) = mvr\hat{n}\sin\theta \end{equation} where $m$ is the mass being considered. The magnitude of the angular momentum is clearly $mvr\sin\theta$ where we are now considering the magnitudes of $\vec{v}$ and $\vec{r}$. Kepler's Second Law has demonstrated that $ k = \frac{rv\sin\theta}{2}$, and thus: \begin{equation} 2km = mvr\sin\theta = |\vec{L}| \end{equation} Since the mass of any planet remains constant around the orbit, we have shown that the magnitude of the angular momentum is equal to a constant. Thus Kepler's Second Law demonstrates that angular momentum is conserved for an orbiting planet.